Electrochemical energy storage systems are the most traditional of all energy storage devices for power generation, they are based on storing chemical energy that is converted to electrical energy when needed. EES systems can be classified into three categories: Batteries, Electrochemical capacitors and fuel Cells. (Source: digital-library.theit.org)
Battery energy storage systems (BESS)
Electrochemical batteries consist of electrochemical cells that convert stored chemical energy into electrical energy. (Source: energyfaculty.com)
Rechargeable batteries (secondary batteries)
Rechargeable batteries are one of the oldest technologies for electrical energy storage (EES) systems, they are extensively used for daily needs and in numerous industrial applications. Electrochemical batteries are made of electrochemical cells that consist of electrodes made up of different types of metals that are submersed into liquid or solid electrolytes.
The basic functioning principle of electrochemical batteries is as follows:
A flow of electrons travels from the negative electrode (anode) to the positive one (cathode), until the anode runs out of electrons. In the case of primary batteries, the process ends here. Whereas for secondary batteries that are rechargeable, this reaction can be reversed using a charger. The electrons will flow from the cathode back to the anode, restoring the initial excess of electrons in the anode. (Source: mdpi.com, Panasonic-eneloop.eu)
The flow of electrons originates from reduction-oxidation chemical reactions that occur in the electrochemical cell; these are commonly called redox reactions and in the case of an electrochemical cell, one half-reaction occurs in each electrode.
Oxidation is the loss of electrons and is the reaction that occurs in the anode, the electrode reacts with the electrolyte producing electrons and we say that the anode is oxidized. Whilst, reduction is the gain of electrons, and is what occurs simultaneously in the cathode, enabling it to accept electrons and we say that the cathode is reduced.
During the discharge cycle, the positive electrode is the cathode and the negative electrode is the anode, electrons flow from the anode to the cathode generating electricity. Whereas, during the charging cycle the reverse reaction happens and the positive electrode is the anode and the negative electrode is the cathode. As shown in the following figure. (Source: mdpi.com)
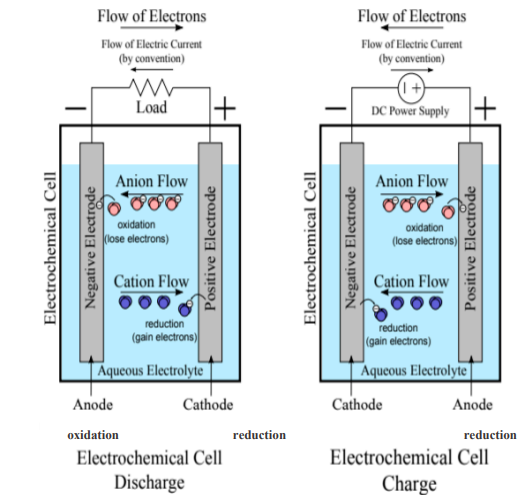
Types of rechargeable batteries
Depending on their chemistry, we can distinguish the main following types of rechargeable batteries.
Lead-acid (PbA) batteries
Lead-acid batteries are the oldest type of rechargeable batteries and one of the cheapest most available solutions that are widely used in automotive sector, industrial applications and power storage systems. They are highly recyclable, their chemistry is considered mature, robust and well understood and are considered one of the most technologically developed and commercially successful battery systems. (Source: mdpi.com, adb.org)
In a Pb-A battery cell, PbO2 is used as the cathode and Pb as the anode and sulfuric acid is used as the electrolyte. The redox reactions that occur in both the anode and the cathode are: (Source: mdpi.com)
The following tables give types of lead acid batteries and their use.
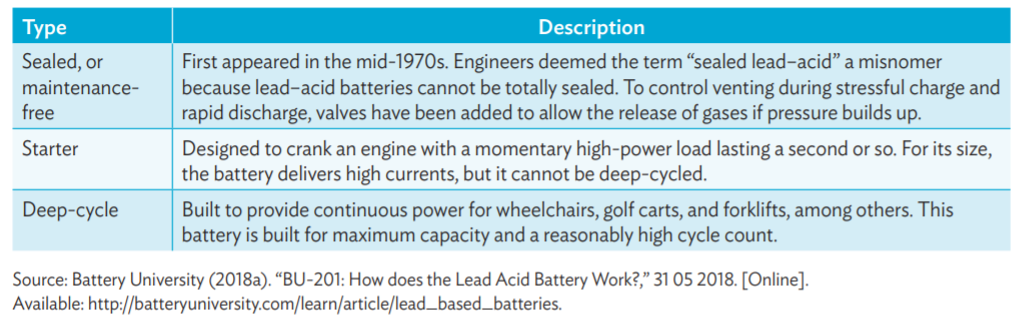
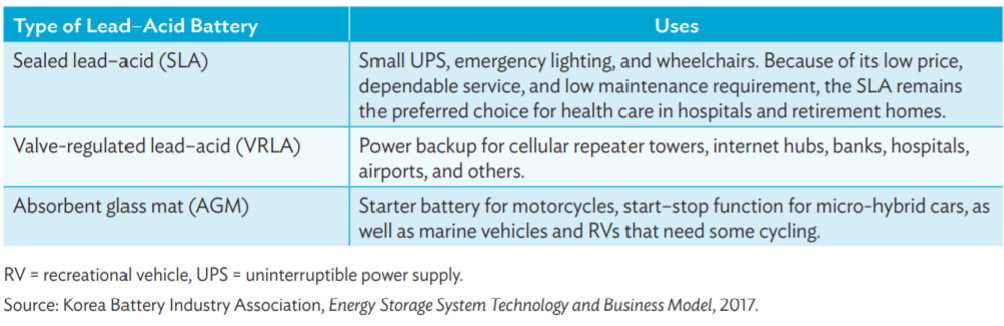
Lithium-ion (Li-ion) batteries
Lithium-ion batteries are the most frequently used electrical energy storage technology in a large range of commercial applications, especially the portable electronic sector. In this type of batteries, the materials used for the anode can be Graphite (LiC6) Li4Ti5O12, Cu, for the cathode LiCoCO2, LiMn2O4 LiNiO2 and electrolytes can be ECM c Propylene carbonate LiPF6, LiBF4, LiClO4. (Source: mdpi.com)
The following table gives Lithium-ion batteries types. (Source: Battery University (2018c))
Types | Description |
Lithium cobalt oxide (LiCoO2) | Made of a cobalt oxide cathode and a graphite carbon anode. Drawbacks of Li–cobalt batteries are their relatively short life span, low thermal stability, and limited load capabilities. |
Lithium manganese oxide (LiMn2 O4 ) | The architecture of this battery forms a three-dimensional spinel structure that improves ion flow on the electrode, resulting in lower internal resistance and improved current handling. These batteries have high thermal stability and enhanced safety, but the cycle and calendar life are limited. |
Lithium nickel manganese cobalt oxide (LiNiMnCoO2, or NMC) | One of the most successful Li-ion systems is a cathode combination of nickel– manganese–cobalt (NMC). Similar to Li–manganese, these systems can be tailored to serve as energy cells or power cells |
Lithium iron phosphate (LiFePO4 ) | Li–phosphate has good electrochemical performance with low resistance. The key benefits are high current rating and long cycle life, besides good thermal stability, enhanced safety, and tolerance if abused. |
Lithium titanate (Li4 Ti5 O12) | Li–titanate replaces the graphite in the anode of a typical lithium-ion battery. The cathode can be lithium manganese oxide or NMC. Li–titanate has a nominal cell voltage of 2.40 V, can be fast-charged, and delivers a high discharge current. |
Nickel-Cadmium (Ni-Cd) batteries
Nickel-cadmium batteries (Ni-Cd) are utilized for portable computers, drills, camcorders, and other small battery-operated devices. Nickel-cadmium batteries can be found in two distinctive designs, a completely sealed cell and a vented cell to release gases (oxygen and hydrogen). Sealed cells are used in daily life whilst vented Ni-Cd batteries are used in aircraft and diesel engine starters, where large energy per weight and volume are critical. (Source: adb.org, mdpi.com)
Reactions occurring at the anode and the cathode are: (Source: mdpi.com)
Nickel–Metal Hydride (Ni–MH) Battery
Ni–MH batteries outperform other rechargeable batteries, and have higher capacity and less voltage depression. These batteries are largely used in high-end portable electronic products, in which battery performance parameters, notably run time, are major considerations in the purchase decision. (Source: adp.org)
Reactions occurring at the anode and the cathode are: (Source: mdpi.com)
Sodium-Sulfur (Na-S) batteries
A sodium–sulfur battery is a type of molten-salt battery made from liquid sodium (Na) and sulfur (S). These batteries have a high energy density, high efficiency of charge/discharge (89–92%) and long cycle life. But they also have some main drawbacks that are their high operating temperatures, ranging from 300 to 350 °C and the highly corrosive nature of the sodium polysulfides. These batteries are primarily suitable for large-scale non-mobile applications, such as grid energy storage. (Source: adp.org)
Flow batteries energy storage (FBES)
Flow batteries or redox flow batteries (RFB) are a category of electrochemical energy storage devices consisting of two liquid electrolyte tanks connected to a cell stack separated by an ion selective membrane. Electrolytes are drawn from the tanks to the cell stack where the process is based on reduction-oxidation reactions of the electrolyte solutions to achieve charging and discharging of batteries. During the charging process, PV panels, wind turbines, or grid input is used to provide electrons to recharge the electrolyte. In the discharging process, the liquid electrolyte is pumped through electrodes to extract the electrons and to generate electricity.
Several types of electrolytes have been used in flow batteries such as zinc (ZnBr), sodium (NaBr), vanadium (VBr) and, more recently, sodium polysulfide. (Source: sciencedirect.com, mdpi.com)
The following figure illustrates a flow battery.
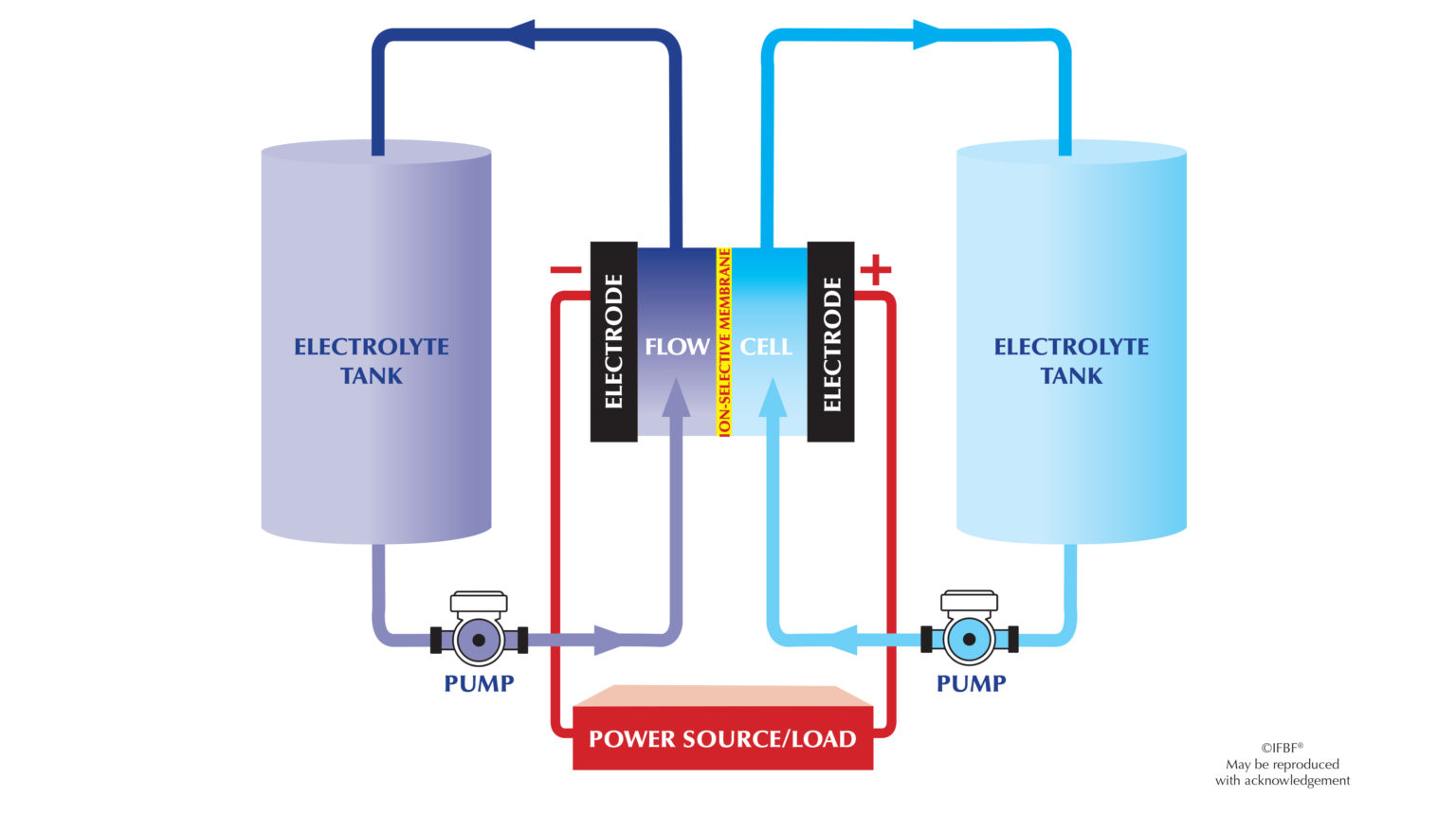
Flow batteries technology is well established and proven to be ideal for long-duration energy output with very low degradation of components within larger, utility-scale deployments and life spans reaching up to 30 years, depending on the electrolyte chemistry. Flow batteries can overcome the limitations of standard electrochemical accumulators (lead–acid or nickel–cadmium for instance) in which the electrochemical reactions create solid compounds that are stored directly on the electrodes on which they form, making them a limited-mass system, that limits the capacity of standard batteries. (Source: powermage.com, sciencedirect.com)
Fuel cell energy storage (FCES)
Fuel cells are devices that convert stored chemical energy into electrical energy. Unlike batteries, these systems do not run down or need recharging since they produce electricity and heat as long as fuel is supplied. FCs function by transforming chemical energy that is stored within whatever energy source such as hydrogen, gasoline or methane, directly into electricity through two electrochemical reactions, making this process non-polluting and about three time more efficient than fuel burning. (Source: energy.gov, energymatters.com.au)
A fuel cell consists of two electrodes (anode and cathode), that are sandwiched around an electrolyte. A fuel is fed to the anode and an oxidant is fed to the cathode. Most fuel cells are powered by hydrogen, which can be fed to the fuel cell system directly or can be generated within the fuel cell system by reforming hydrogen-rich fuels such as methanol, ethanol, and hydrocarbon fuels. In a hydrogen fuel cell, hydrogen is fed to the anode and oxygen to the cathode, a catalyst at the anode separates hydrogen molecules into protons and electrons. The electrons go through an external circuit, creating a flow of electricity and the protons migrate through the electrolyte to the cathode, where they unite with oxygen and the electrons to produce water and heat. (Source: energy.gov, energymatters.com.au)
A hydrogen fuel cell is illustrated in the ‘’Hydrogen and decarbonization” page. (Click the on the word ‘’Hydrogen and decarbonization’’ to consult it)
Types of fuel cells
Fuel cells are classified primarily by the kind of electrolyte they employ, these types are given in the following table. (Source: energy.gov)
Fuel cell type | Description |
Polymer electrolyte membrane fuel cells | PEM fuel cells (also called proton exchange membrane fuel cells) utilize a solid polymer as an electrolyte and porous carbon electrodes containing a platinum alloy catalyst. They function using hydrogen, oxygen from the air, and water and require relatively low temperatures of about 80°C. PEM fuel cells are used primarily for transportation applications (vehicle applications, such as cars, buses, and heavy-duty trucks.) and some stationary applications. |
Direct methanol fuel cells | DMFCs, are powered by pure methanol, which is usually mixed with water and fed directly to the fuel cell anode. DMFCs are often used to provide power for portable fuel cell applications such as cell phones or laptop computers. |
Alkaline fuel cells | AFCs use a solution of potassium hydroxide in water as the electrolyte and can use a variety of non-precious metals as a catalyst at the anode and cathode. In recent years, novel AFCs that use a polymer membrane as the electrolyte have been developed. These fuel cells are closely related to conventional PEM fuel cells, except that they use an alkaline membrane instead of an acid membrane. These cells have demonstrated efficiencies above 60% in space applications. |
Phosphoric acid fuel cells | PAFCs use liquid phosphoric acid as an electrolyte and porous carbon electrodes containing a platinum catalyst. These are considered the first generation of modern fuel cells, they are typically used for stationary power generation, but some PAFCs have been used to power large vehicles such as city buses. |
Molten carbonate fuel cells | MCFCs are high-temperature fuel cells that use an electrolyte composed of a molten carbonate salt mixture suspended in a porous, chemically inert ceramic lithium aluminum oxide matrix and are currently being developed for natural gas and coal-based power plants for electrical utility, industrial, and military applications. |
Solid oxide fuel cells | SOFCs operate at very high temperatures and use a hard, non-porous ceramic compound as the electrolyte. They are around 60% efficient at converting fuel to electricity. |
Reversible fuel cells | Reversible fuel cells produce electricity from hydrogen and oxygen and generate heat and water as byproducts, just like other fuel cells. However, reversible fuel cell systems can also use electricity from solar power, wind power, or other sources to split water into oxygen and hydrogen fuel through a process called electrolysis. |
Electrochemical Capacitors (EC)
Whilst a capacitor is an electrical energy storage system that is a two-terminal passive electronic component that stores charge in the form of electric field between its metal plates. A capacitor is constructed of two electrodes (an anode and a cathode), that are separated by an insulator known as a dielectric; common dielectrics include air, oiled paper, mica, glass, porcelain, or titanate. An external voltage difference is applied across the two plates, creating a charging process during which, positive charges gradually accumulate on one plate (positive electrode) and negative charges accumulate on the other (negative electrode). When the external voltage difference is removed, both the positive and negative charges remain at their corresponding electrodes. The voltage difference between the two electrodes is called the cell voltage of the capacitor. If these electrodes are connected using a conductive wire with or without a load, a discharging process occurs and the positive and negative charges will gradually combine through the wire and the capacitor will play a role of charge storage and delivery. An electrochemical capacitor, often referred to as electric double-layer capacitor (EDLC), supercapacitors or ultracapacitor, is an electrochemical energy storage device exploiting charge accumulation in an electric double-layer at the interface between a high-surface-area carbon electrode and a liquid electrolyte. (Source: electricaltechnology.org, hal.archives-ouvertes.fr)
The following figures illustrate an electrochemical capacitor and a conventional capacitor.
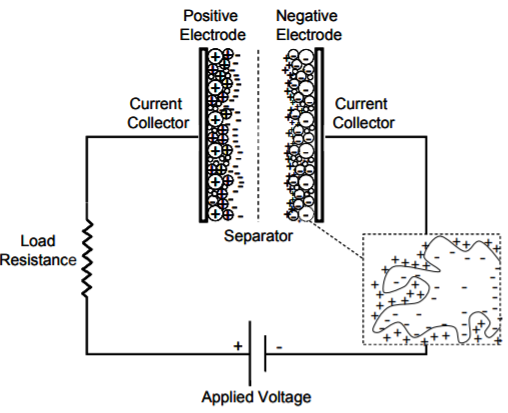
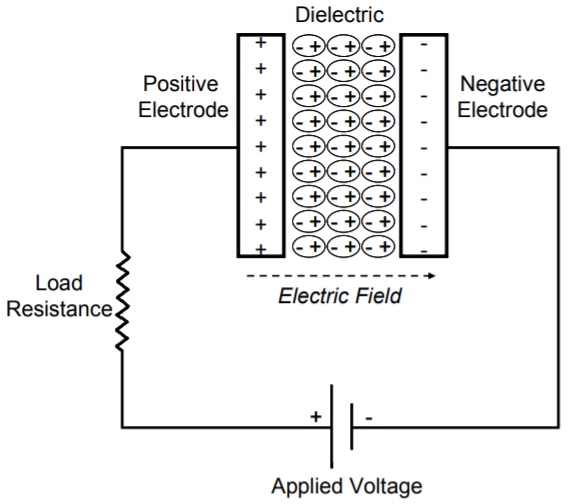
Supercapacitors store charge either using electrostatic double-layer capacitance (EDLC) or electrochemical pseudo-capacitance or both known as hybrid capacitance. (Source: electricaltechnology.org)
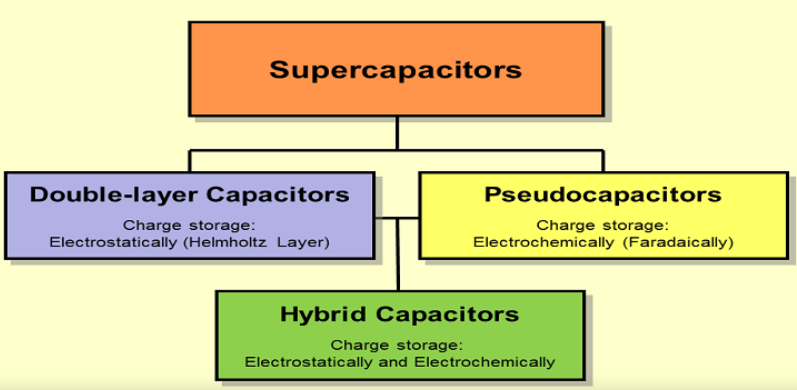
Electrochemical Double-Layer capacitors
Electrochemical double-layer capacitors (EDLCs) are constructed from two carbon-based electrodes, an electrolyte, and a separator and they utilize an electrochemical double-layer of charge to store energy. As a conventional capacitor, in EDLCs there is no transfer of charge between electrode and electrolyte and charge is stored electrostatically (non-Faradaically).
When voltage is applied, charge accumulates on the electrode surfaces. Following the natural attraction of unlike charges, ions in the electrolyte solution diffuse across the separator into the pores of the electrode of opposite charge. However, the electrodes are engineered to prevent the recombination of the ions. Thus, a double-layer of charge is produced at each electrode, as shown in the following figure. These double-layers, coupled with an increase in surface area and a decrease in the distance between electrodes, allow EDLCs to achieve higher energy densities than conventional capacitors. (Source: mitre.org)
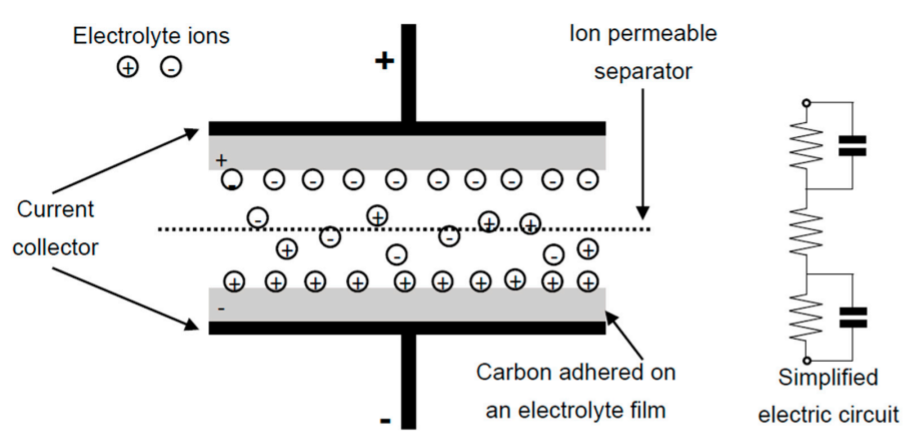
Pseudocapacitors
Pseudocapacitors store charge Faradaically through the transfer of charge between electrode and electrolyte. This is accomplished through electrosorption, reduction-oxidation reactions, and intercalation processes. These Faradaic processes may allow pseudocapacitors to achieve greater capacitances and energy densities than EDLCs. (Source: mitre.org)
(Capacitance being the defined as the ratio of stored (positive) charge Q to the applied voltage V )
Hybrid capacitors
Hybrid capacitors employ both Faradaic and non-Faradaic processes to store charge, they have higher operating voltage (3.8 V maximum) and much higher capacitance and energy density (up to 10 times) than supercapacitors and they also have much lower self-discharge and standby current. (Source: eaton.com)
Electrochemical capacitors have relatively high-power densities, but relatively low energy densities when compared to electrochemical batteries and to fuel cells. A battery can store more total energy than a capacitor, but it cannot deliver it very quickly, which means its power density is low. Capacitors, on the other hand, store relatively less energy per unit mass or volume, but can discharge it rapidly to produce a lot of power, so their power density is usually high. (Source: mitre.org)
The following figure gives comparison of batteries, ECs and fuel cell energy and power density. (Source: sciencedirect.com)
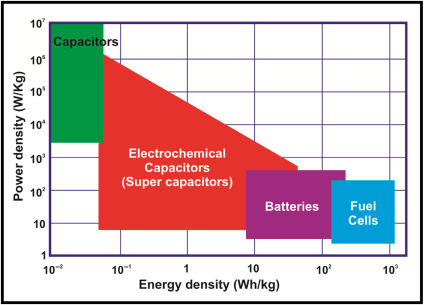
Electrochemical energy storage data
According to Statista’s operational electrochemical storage power capacity in the EU 2020, by country article, the United Kingdom has the highest power capacity of operational electrochemical storage facilities in European countries, at 570 megawatts. With the UK formally leaving the European Union in January 2020, Germany is currently the EU member state with the highest operational electrochemical storage power capacity, at 406 megawatts. (Source: statista.com)
The following figure gives power capacity of operational electrochemical storage plants in the European Union as of 2020.
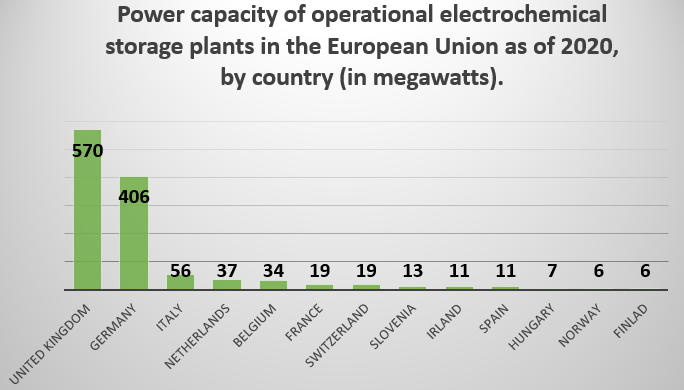
According to Marketsandmarkets, the global fuel cells market size is expected to reach USD 848 million by 2025 from an estimated value of USD 263 million in 2020, growing at a CAGR of 26.4%. The market has been analyzed with key focus on advancements in fuel cell technologies such as PEMFC, PAFC, AFC, and MFC. The growth is due to the rising demand for clean energy generation in developed regions, increased use of fuel cell-based vehicles, booming power sector, and augmented power generation capacities globally. (Source: marketsandmarkets.com)
According to Battery Energy Storage System, Update 2021 – Global Market Size, Competitive Landscape, Key Country Analysis to 2025 report, Asia-Pacific (APAC) was the largest market for battery energy storage systems in 2020, accounting for 49.9% of the global market installed capacity. The region is forecast to maintain its top position in the forecast period up to 2025, as well as its market share of approximately 53.5% in 2025, followed by Europe, Middle East and Africa (EMEA) and the Americas. (Source: store.globaldata.com)
According to Global Supercapacitor Market with COVID-19 Impact Analysis by Type, Electrode Material, Application, Region – Global Forecast to 2025 report, the Supercapacitor market is projected to grow from USD 409 million in 2020 to USD 720 million by 2025; it is expected to grow at a CAGR of 12.0% from 2020 to 2025. This growth is driven by their increasing demand on energy harvesting applications and rising use of supercapacitors in trains and aircraft. Moreover, the increasing global demand for electric vehicles is likely to fuel the growth of the market. (Source: marketsandmarkets.com)